Such a system, i.e., an atom, can decrease its energy by the transition of the
electron into an energetically lower orbit. Since such a transition is a pure quantum mechanical process, each, in this sense, quantum mechanically not forbidden but "allowed" transition causes the emission of radiation energy in the form of photons of a certain frequency and energy.
Thus, the spectra of an atom are very characteristic for that particular atom.
The c quarks of the charmonium system are bound together by means of the strong force. The relative motion of the bound quarks gives rise to the orbital angular momentum, measured in whole-number multiples of Planck’s constant h, which contributes to the total energy of the system. The quarks, like the proton and electron, also possess some intrinsic angular momentum called spin. Quantization of these angular momenta results in a unique set of allowed energy levels for the charmonium system in analogy to those of an atomic system.
In this context, a charmonium state is often referred to as "hydrogen atom of strong interaction".
All Charmonium states are heavier than a hydrogen atom which has a mass of roughly 0.94 GeV/c2 or 1.67 •10-27kg.
The charm quark c, discovered in 1974, has a mass of approximately 1.3 GeV/c2 or 2.6 • 10-27 kg. The lightest charmonium state, the ηc meson, has a mass of 2.98 GeV/c2.
Unlike the hydrogen atom or other stable matter, the J/ψ meson and all
charmonium states are unstable particles and decay. That means that even a charmonium ground state is not stable whereas a hydrogen atom in its ground state should exit forever.
The J/ψ meson, for instance, has a mean lifetime on the order of 10-21 s.
All chamonium states, can, in principle, decay into
• other hadrons via the strong interaction
• into leptons via the electromagnetic interaction
Although the charmonium states predominantly decay via the strong interaction into hadrons, additionally the excited charmonium states can decay into lower energy states by the emission of a photon, just as in atomic physics.
Thanks to this result, the LHCb collaboration opens a new avenue to precision measurements of charmonium particles at hadron colliders, that was unexpected by the physics community. - Giovanni Passaleva, Spokesperson for the LHCb collaboration
Analogous to the hydrogen atom, a c-quark c-antiquark pair (cc) in a bound state must occupy discrete energy levels as well. The ηc meson represents the charmonium ground state with spin 0 (zero). Each of its constituents, the c-quark and the c-antiquark are particles with spin ½. Therefore, the lowest energy state corresponds to an antiparallel spin-vector orientation which results in a spin magnitude of 0 for the composite particle system.
In its first excited state, the spin-vectors of both c quarks are aligned parallel so that their magnitudes sum up to spin 1. The first excited state of a charmonium is called a J/ψ (J/psi) meson which is a spin 1 particle state.
To reach energetically higher states, the system, i.e., the J/ψ meson has to be excited again, which means the system must absorb energy which is stored as potential energy of the two quarks occupying 'higher' orbits. Two of those excited states are represented by the χc1 and χc2 mesons.
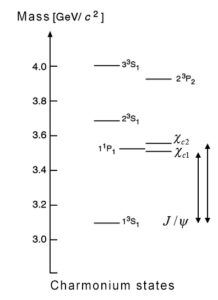
The plot shows a part of the spectrum of photon energies observed when in an excited J/ψ meson one of the excited energy states relaxes to lower-energy levels. As we see here, the mass of the c quark- c antiquark pair in an excited ψ state is higher than the sum of masses of its constituents which means that the system has stored binding energy. This stored energy has a mass equivalent, because of E/c² = m.
The LHCb experiment studied, for its first time, the particular transformation of χc1 and χc2 mesons decaying into a J/ψ particle and a pair of muons in order to determine some of their properties very precisely. Previous studies of χc1 and χc2 at particle colliders have exploited another type of decay of these particles featuring a photon in the final state instead of a pair of muons.
However, measuring the energy of a photon is very challenging experimentally in the harsh environment of a hadron collider. This new measurement opens a new avenue to precision studies of the properties of χc mesons at the LHC, more than 40 years after the November Revolution took place.
As Giovanni Passaleva stated:
“Not only are we no longer obliged to resort to purpose-built experiments for such studies, but also, in the near future, we will be able to think about applying a similar approach for the study of a similar class of particles, known as bottomonium, where charm quarks are replaced with beauty quarks.”
For an easy introduction into particle physics and the standard model, check out also our latest e-book edition: "The Mystery Of The Higgs Boson".